Volatility: The V in CVD
In order to deposit a material using a chemical vapor deposition process, it must be possible to form a precursor -- a chemical which can be converted into the desired solid material but which is itself either a gas, or easily converted into a gas at a modest temperature. What determines the vapor pressure of a material?
Equilibrium Vapor Pressure
The concentration of a vapor which is in equilibrium with the liquid -- the vapor pressure of the substance -- is determined, like the equilibrium of any other simple reaction taking place at constant total pressure, by the change in free enthalpy ("Gibbs free energy") G = H - T*S, where H is the enthalpy (U + PV) and S is the entropy. The concentration is denoted by brackets: [A] is the concentration of substance A relative to the "standard state", typically P = 1 atmosphere, T = 25 °C.
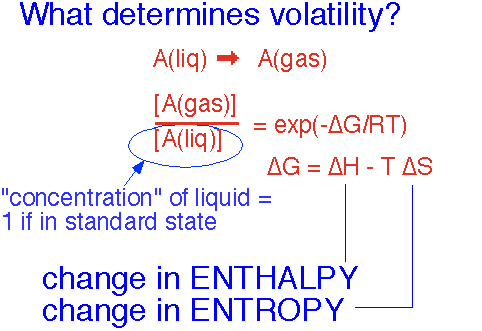
Thus the free energy change in going from the liquid to the gaseous state determines the concentration of the gas -- i.e., the vapor pressure.
Recall that entropy is essentially proportional to the logarithm of the number of states accessible to a system. The change in S upon evaporation is dominated by the increase in the number of states accessible to molecules when they are in gaseous form instead of within a liquid or solid, with a modest influence from the degree of order in the solid or liquid. Since this term is multiplied by the temperature, entropy becomes more important as the temperature rises-- the commonplace observation that evaporation occurs more readily when an object heats up.
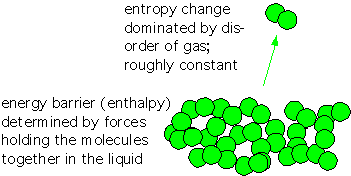
The change in enthalpy H is mostly determined by the strength of the forces which hold the atoms or molecules in the liquid or solid state. Let’s take a look.
Intermolecular Forces
Most CVD precursors evaporate in molecular form from a molecular liquid or solid. The change in enthalpy upon evaporation is determined by the forces which hold the molecules together. We'll review them, from the weakest to the strongest.
Van der Waals forces
These forces between neutral molecules arise from the tendency of the electrons in nearby molecules to fluctuate in a correlated fashion. The strength of the force varies as the inverse sixth (!) power of the distance, so van der Waals forces are only important at very short ranges.
The strength of van der Waals interactions is essentially proportional to the number of electrons which interact. The van der Waals force between atoms increases roughly as the square root of the atomic number. The attraction between molecules increases about linearly with the number of atoms in the molecule if the molecule is linear or flat; "compact" molecules with less surface area have correspondingly less van der Waals attraction.
Van der Waals force for inert gases (J/mole):
He-He 76
Ar-Ar 1050
Xe-Xe 1990
Van der Waals force for some organic compounds (estimated from boiling point, again J/mole):
CH4 9300
C5H12 (pentane) 25,500
Hydrogen bonding
Certain electronegative atoms (O, N, and F) when incorporated into a molecule can still form fairly strong bonds with nearby hydrogen atoms attached to another electronegative atom. Hydrogen bonds are partially electrostatic in origin: the hydrogen attached to e.g. O has a partial positive charge, the electronegative atom a partial negative charge. Typical bond strength is about 20 KJ/mole/bond, so they are on the order of 10-20 times stronger than the van der Waals attraction per bond.
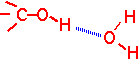
Covalent bonding
We have already discussed the strong bonds between atoms ("covalent bonds"), which occur due to the rearrangement of the valence electrons to minimize overall energy. Covalent bonds are generally much stronger than hydrogen bonds or van der Waals forces. When a substance is held together by covalent bonds (e.g. a silicon crystal) it will be very difficult to cause atoms to evaporate from the surface.
Typical covalent bond energies (kJ/mole):
C-H 320-400
C=C 600-700
O-Br 200
Cl-N 120
Transition-metal bonding; Coordination complexes
We saw in our discussion of covalent bonding in simple first-row elements that up to four bonds could be constructed out of the four valence orbitals (s, px, py, pz). However, in CVD we are often interested in deposition of transition metals, elements lower on the periodic table which have d or f electrons in the valence shell, and can thus take higher coordination numbers (that is, bond to more atoms) than allowed by s-p bonding. In order to create volatile materials with such atoms, we must avoid forming chains of such bonds holding the molecules together. This is a particular problem in the bottom left corner of the periodic table (Ba, Sr, La), where the atomic "diameter" is large.
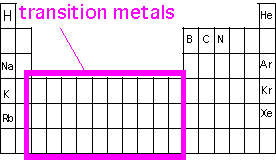
We can solve this problem in several ways. We can use highly electronegative substituents like F or Cl to make strong bonds with the active atom and surround it, preventing other atoms from bonding. However these strong bonds will require lots of energy to break, raising the deposition temperatures. The carbonyl moiety CO is also used in this fashion. Alternately, we can use large ligands with inert exteriors to physically surround the active atom and prevent bonding: acetylacetonates are members of this class of materials.
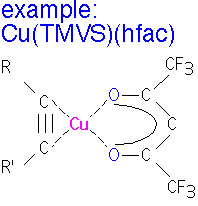
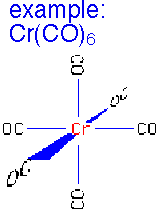
We can conclude that to form a volatile precursor molecule, we must find substances that form molecules with modest surface area (to minimize van der Waals forces), avoid hydrogen bonding between molecules, and avoid the formation of covalent bonds in the liquid or solid state.
Entropy: Trouton’s Rule
Recall that the change in free energy which determines the vapor pressure also has a contribution from the change in entropy. Below we show a sample of entropies of vaporization for a variety of substances which are reasonably volatile around room temperature.
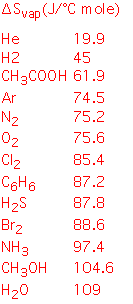
We can summarize the data as a histogram.
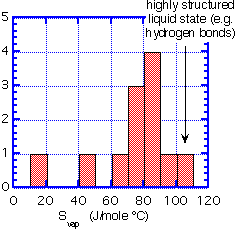
We see that the majority of substances have an entropy of vaporization of 70 to 90 J/mole degree. This empirical observation is Trouton's rule. It fails for very small, light molecules (He and H) or for substances which have strong hydrogen bonding in the liquid state (water).
The vapor pressure of a gas at its boiling point, 1 atmosphere, is equal to its "standard state". The "concentration" is therefore 1, so the change in free enthalpy G upon vaporization must equal 0: both the liquid and gas are in their standard states. This means that the change in enthalpy divided by the boiling temperature is equal to the change in entropy, that is about 85 J/mole degree. Thus if we know the boiling temperature we can come up with a rough estimate of the enthalpy of vaporization as well.

If we know both the enthalpy and entropy of vaporization, we can derive the vapor pressure at any temperature (rather approximately) by using the Clausius-Clapeyron equation. In particular, we can use the boiling point for To and get the vapor pressure in atmospheres.
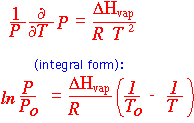
Examples of Volatile Precursors
Silicon
The most common precursor for silicon and related compounds is silane, SiH4. This is a small molecule, and should have little van der Waals attraction. It should have little dipole moment both because the Si-H bond is not very polar, and because of the symmetry of the molecule. There is nothing to hydrogen-bond to, and the silicon covalent bonds are saturated. Thus it would be expected to be highly volatile, and in fact silane is a gas at room temperature even under pressure (boiling point -112 °C).
Disilane, Si2H6, is a larger molecule and thus less volatile; its boiling point is -14 °C, so it can be liquified under pressure at room temperature.
Another common precursor molecule is dichlorosilane, SiH2Cl2. The larger chlorine atoms have higher electron density than hydrogen, making this molecule less volatile, with a boiling temperature of 8 °C.
SiO2
In addition to the various silanes above, we can use precursor molecules in which oxygen-silicon bonds already exist, such as TEOS, Si(OC2H5)4. This material has a larger surface area due to the four ethyl (C2H5) groups on the outside; the vapor pressure of TEOS is only around 5 Torr at room temperature, with a boiling point of about 160 °C.
Tungsten
The most common precursor for deposition of tungsten is tunsten hexafluoride, WF6. Here the tungsten is surrounded by six strongly electronegative F atoms. The outer electron configuration of the W atom has 4 d electrons and 2 s electrons, so all six electrons are used up in the bonds. The boiling point of WF6 is about 17 °C: it is a highly volatile liquid at room temperature.
Note that the vapor pressure of WF6 is comparable to that of dichlorosilane, even though the molecular weight is much larger: 298 vs. 101. It is not the mass of the molecule but the intermolecular forces that primarily determine volatility.
The Numbers Please: boiling points...
SiH4 -112°C
Si2H6 -14°C
SiH2Cl2 8 °C
WF6 17°C
TEOS 160°C
Some general conclusions:
- No Covalent Bonds
- Saturated outer shells: SiF4, SiCl4, TiCl4, WF6
- Small is better
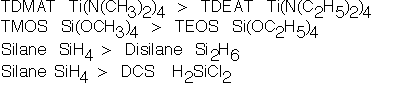
- "Big atoms, Big problems"
Hard cases: Ba, Sr, La. Thus difficult applications are: superconducting materials, high-K dielectrics, electro-optic films.
Return to Tutorial Table of Contents
Book version of the CVD Tutorial