Moisture Barrier Effects in Deposited Oxides
Summary
In order to improve planarity of intermetal dielectrics, both spin-on glasses (SOG's) and relatively conformal oxides from TEOS rather than SiH4 precursors were employed in IC manufacturing (until Copper Damascene processing became dominant). However, these materials have typically incorporated larger amounts of silanol (Si-OH) and adsorbed water than the SiH4 - based oxides previously used. The total amount of intermetal dielectric has also increased as the number of metal layers increases. As a result, the lifetime of the underlying transistors under high-field stress is reduced after deposition of the intermetal dielectrics.
To alleviate this and related problems, several workers have reported the use of moisture barrier films deposited beneath the interlayer dielectrics. These films have been deposited both by conventional plasma-enhanced CVD, and by high-density (ECR) plasma, from SiH4 with N2O, O2, and N2. In all cases, effective "barriers" are higher in refractive index than typical SiO2, and have either excess Si or N incorporation. Appropriately prepared films completely eliminate degradation in hot-carrier lifetime due to intermetal dielectric deposition and anneal.
The work of Machida et. al. at NTT in particular has provided strong evidence that these films are not in fact barriers, but more properly traps for moisture. They have shown that Si-H bonds are essential for operation of the films, and that H2 evolution from these films is strongly enhanced if they overlie a "wet" film such as TEOS/O3 oxide, suggesting that the mechanism is:
=Si-H + H2O => =Si-OH + H2
(where "=Si" denotes the bonds to the rest of the silica lattice) and that in the presence of excess Si-H bonds the subsequent reaction
=Si-H + =Si-OH => =Si-O-Si= + H2
can result in complete removal of moisture without forming water or leaving silanols in the film.
Literature Review
The Problem:
When operated at high drain voltages, MOSFETs generate "hot" electrons near the drain (see figure 1).
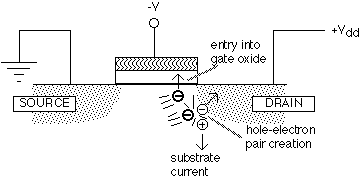
Fig. 1: Effects of hot electrons in a MOSFET (after [13])
Some of these hot electrons gain enough energy to enter the oxide conduction band (about 3 eV above the silicon conduction band). While traveling through the oxide, a small fraction of the electrons are trapped, giving rise to undesirable changes in the transistor characteristics. Other hot electrons generate hole-electron pairs: the generated electron is attracted to the positive drain, while the hole is repelled and typically moves into the substrate, causing a measurable substrate current. It is found empirically that the time required to induce a change in the transistor characteristics is inversely related to the substrate current, unsurprising since the same hot electrons are responsible for both phenomena. In consequence, the reliability of a MOSFET under high drain voltage (hot electron stress) is often measured by plotting the lifetime versus the substrate current on a log-log scale.
The ability of the gate oxide to tolerate these stresses varies widely, and depends not only on gate oxide growth conditions, but also on back-end processing. Many workers reported hot carrier reliability problems due to components of the intermetal dielectric. Some examples are shown in figure 2.
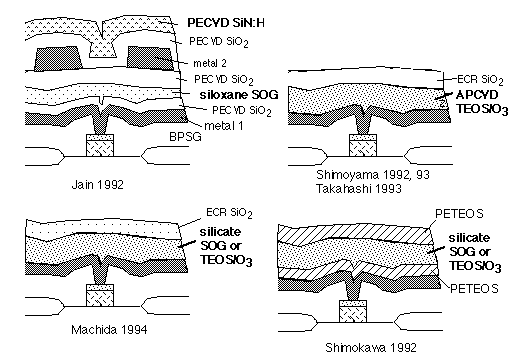
Fig. 2: Structures causing degraded hot-electron lifetime of underlying MOSFETs (culprits labeled in boldface)
In every case [1-6], the putative culprits are SOGs or TEOS/O3 films: known carriers of Si-OH and water, and subject to pickup of water vapor on ambient exposure, as verified by direct measurements of desorbed water vapor on heating [2,3,5]. Thus we can assume that water or hydroxyl released during deposition or annealing steps diffuses to the underlying gate oxides, where it enhances electron trapping and reduces lifetime.
Solutions and a Mechanism
The deleterious effects of a water-containing dielectric can be avoided by interposing an appropriate "barrier" layer between the wet layer and the underlying FETs. Layers that are reported to work well are summarized in table 1.
Table 1: Effective Underlayers to Avoid Hot Carrier Reliability Degradation
Film type
Reference
PECVD oxide from SiH4 with high Si-H bond content
[1,14] ECR oxide with high Si-H bond content
[2,4,5,6] ECR oxynitrides
[8] dual frequency PECVD oxide or oxynitride from SiH4/N2O, n>1.5
[10] PETEOS oxides with high O2:TEOS ratio (at least for TEOS/O3)
[14]
Machida et. al. [6] have performed a very thorough study of their barrier layers, placed under a sandwich containing both silicate SOG and APCVD TEOS/O3 oxide. The barriers were deposited by ECR from SiH4 and O2 with varying ratios of gas flows, at temperatures "below 200°C". They showed:
a) hot-electron reliability is determined by the SiH4:O2 ratio of the ECR "barrier" oxide
b) with increasing SiH4:O2 ratio, FTIR shows shift from Si-OH to Si-H bonds (and some SiH2 at higher ratios)
c) measurements of thermally-stimulated desorption (TSD) from SOG covered by ECR oxide not only showed suppression of H2O but also an increase of H2 over the sum of the two films alone. SiH4:O2 = 0.5 delayed water release until T>450°C; SiH4:O2 = 0.7 completely eliminated desorption of water up to 900°C.
These observations are summarized graphically in figures 3 and 4.
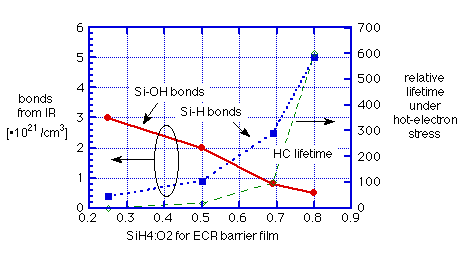
Fig. 3: Effect of gas mixture on hydrogen incorporation and barrier effectiveness for ECR oxide: data of Machida et. al. [6]
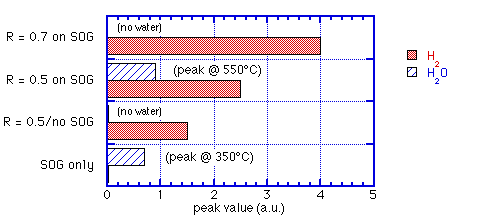
Fig. 4: Peak H2 and H2O released for varying ECR/SOG sandwiches: data of Machida et. al. [6]
The mechanism proposed to explain the data is:
1) Si-H bonds react with water to form silanol, with release of molecular hydrogen gas:
=Si-H + H2O => =Si-OH + H2
2) In the presence of excess Si-H, the silanols may be consumed by:
=Si-H + =Si-OH => =Si-O-Si= + H2
Both reactions are needed to explain the increased hydrogen release of the combined films, and the complete suppression of water evolution for high Si-H concentrations. Furthermore, this mechanism is quite consistent with observations of other authors:
Jain et. al. [1] also observed a direct dependence of hot-carrier lifetime on Si-H bond concentrations in the barrier layer, as shown in figure 5:
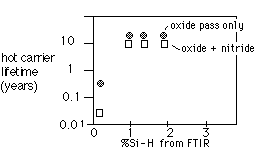
Fig. 5: Hot carrier lifetime for PECVD-SOG IMD vs. %Si-H in PECVD layer [after Jain et. al. [1]]
Shimokawa et. al. [14] observed that the IR spectra of PECVD "barrier" oxides deposited at 1.5 Torr, which gave good hot carrier lifetime in sandwiches with SOG, displayed a significant SiH2 peak at 880/cm and shift of the Si-O-Si stretch to lower wavenumbers. (Unfortunately, these authors did not show the region around 2100/cm which shows Si-H bonding.) Similar films deposited at 2.5 Torr showed no indication of Si-H or Si-rich features, and resulted in poor hot carrier reliability.
Doki et. al. [9] also found that ECR oxides grown from SiH4-rich mixtures provided excellent resistance to moisture penetration. Unfortunately, again these authors provide no IR data in the region around 2100/cm where Si-H is most evident.
Does the Method Matter?
Good moisture barrier properties have been obtained both with ECR-based oxides and nitrides, and with conventional PECVD depositions. In ECR depositions it appears that simply changing the SiH4:O2 ratio to produce large concentrations of Si-H bonds is sufficient to obtain acceptable films, with good moisture barrier behavior and no undesirable changes in film properties.
Where Does PETEOS Stand?
Shimokawa et. al. reported, as we noted previously, that acceptable barrier performance can be obtained (at least vs. TEOS-O3 films) using a PETEOS film deposited with lots of O2. No data was given on Si-H content, but the optimal film was shown to have much less Si-OH and H2O incorporation (from IR absorption around 3200 -3700 /cm) than the non-optimal film. Most other authors have shown PETEOS films to be pretty useless as moisture barriers, though the conditions of deposition are not generally reported. It is well-known that PETEOS films deposited with tensile stress are more likely to acquire moisture from exposure to ambient, and that brief plasma treatments (especially in nitrogen-containing ambients) can reduce the hygroscopic tendencies of thermal TEOS/O3-based oxides. Thus it seems likely that with careful optimization of process conditions, PETEOS oxide layers can provide some benefit.
However, Yamaha et. al. [12] showed that even a carefully optimized PETEOS film that evolved very little water still had a modest but measurable (about 3X) deleterious effect on hot carrier lifetime. Through the use of specialized test structures covered with wide metal lines, they showed that degradation due to a SOG film is predominantly due to a species which diffuses rather slowly in oxide, whereas the PETEOS effect was caused by a much faster diffuser. They suggest that the SOG culprit is molecular water, H2O, while the PETEOS releases the hydroxyl radical OH*.
The situation in conventional PECVD is a bit more complex. Shimokawa et. al. [14] reported good moisture barrier results just by changing pressure in what seems likely to be a Novellus Concept 1 or close relative thereof. Blain et. al. [7], working with a Concept 1, also found that simply changing the pressure of deposition could give a silicon-rich film which was stable in air without stress hysteresis (but unfortunately give no details about their deposition conditions). They report 6% nitrogen and 6% hydrogen by RBS / nuclear reactio profiling, but don't provide IR data, so we don't know what chemical state the hydrogen was in.
Lee et. al. found that high-hydrogen films prepared in an Applied Materials 5000, while excellent moisture barriers, showed excessive stress hysteresis after exposure to ambient unless a dual-frequency operating mode was employed. Standard PECVD uses N2O as an oxidant for SiH4 and results in some nitrogen incorporation; Lee et. al. found that the films with the best combination of moisture resistance and low stress hysteresis contained significant amounts (15-25 at%) of nitrogen. Optimized films suppressed moisture evolution to > 800°C. These authors assert that refractive index is a sufficient measure of moisture barrier capability (n > 1.6 is good) but don't give us enough IR data to establish whether n and Si-H are monotonically related.
We conclude that excellent moisture barriers can be fabricated by conventional PECVD techniques, but that the optimal deposition conditions are not achieved merely by increasing SiH4 concentration as in the ECR case: results can be sensitive to chamber pressure and ion bombardment energy, and incorporation of nitrogen may be helpful.
Doki et. al. directly compared ECR and PECVD oxides with refractive index of 1.5, showing that the ECR oxide had much superior moisture barrier performance. However, Lee's work shows that PECVD oxides with n=1.5 are not necessarily the best films for this purpose: thus the comparison is of an optimized ECR film against what is probably a sub-optimal PECVD film.
The addition of N2 in ECR reactors can be used to deposit oxynitrides. Ishikawa and Murao [8] showed that oxynitrides made excellent barriers to moisture penetration. They claimed that these were significantly superior to ECR oxides in that respect, but it seems they cheated here a bit: the IR data (complete for once!) shows that their N2=0 oxide has negligible Si-H bonding, whereas the oxynitrides have strong Si-H peaks. Thus it is not possible to ascertain whether nitrogen itself plays a role (through e.g. increasing the connectivity of the lattice to reduce the diffusivity of water) or is simply enhancing incorporation of hydrogen into the film.
Extrapolation to Higher Temperatures
In early work [4] the NTT group showed that annealing a barrier oxide prior to adding the "wet" layer could degrade its effectiveness in preventing moisture penetration. At that time the authors ascribed the effect to the removal of ESR-visible defects, but later suggested that these defects play an insignificant role. Unfortunately, the authors at this time did not provide total Si-H after anneal, so it is difficult to reconcile these results with their later work.
The same group demonstrated that hydrogen evolution from Si-H rich ECR oxides is significant from about 300 to 800-900°C. Lee et. al. showed that moisture-blocking PECVD oxynitrides also evolve significant hydrogen to 800°C. In both cases release of water vapor from wet underlying layers is completely prevented by appropriate barriers. Thus we can conclude that "gettering" of water vapor by Si-H in these layers may remain effective up to the temperatures encountered in front-end processes.
Some support for this assertion is provided by the work of Dun et. al. [11]. These authors found that a "silicon-rich" PECVD oxide was necessary as a BPSG underlayer to avoid large shifts in field threshold voltage during flow (950°C?). The post-flow "Si-rich" oxide showed no sign of residual hydrogen. While few details are provided, we find it plausible to guess that this layer was acting to convert water released by the BPSG in the early phases of the anneal to hydrogen, preventing deleterious effects on the underlying thermal oxide.
Metrology
Hot carrier reliability
The only way to measure hot-carrier reliability is to operate transistors under high-voltage stress. This requires specialized test structures containing working transistors with at least one level of interconnect. The actual tests are not unduly sophisticated and can be easily performed using simple semi-automated gear, but the acquisition of enough data to be useful requires automated testing facilities.
Barrier film properties
Composition:
The quantities of hydrogen of interest are easily detected by infrared spectroscopy for reasonable film thicknesses (a few thousand Å). Of particular interest are the size and shape of the Si-H bands around 2100-2300/cm. Auger, ESCA, or RBS can be used to examine the absolute concentrations of Si, O and N.
Moisture barrier properties:
Several authors have employed a nice test structure for this purpose: an unannealed PSG film covered with the barrier in question. This film can then be tested by exposure to moisture in e.g. a pressure cooker at Å 120°C (2 atmospheres H2O). The results are easily evaluated by FTIR: penetration of moisture to the PSG will result in strong reductions of the P=O peak in the infrared spectrum. (It is of course necessary to protect the PSG films from exposure to moisture prior to deposition of the cap.)
Desorption:
It is desirable to monitor both evolved water and H2 from room temperature to about 850°C. Commercial equipment is available to monitor evolution of low-molecular-weight gases from films during heating to high temperatures.
References
1 "Internal Passivation for Suppression of Device Instabilities Induced by Backend Processes", V. Jain, D. Pramanik, S. Nariani and C. Hu [VLSI / UC Berkeley], 1992 Intl Reliability Physics Meeting p. 11
2 "Enhanced Hot-Carrier Degradation due to Water in TEOS/O3 Oxide and Water Blocking Effect of ECR-SiO2", N. Shimoyama, K. Machida, K. Murase and T. Tsuchiya [NTT], Symposium on VLSI Technology 1992 p. 94
3 "Suppression of the MOS transistor hot carrier degradation caused by water desorbed from intermetal dielectric", K. Shimokawa, T. Usami, S. Tokitou, N. Hirashita, M. Yoshimaru, and M. Ino [Oki Electric], 1992 Symposium on VLSI Technology Digest p. 96
4 "Water trapping of point defects in interlayer SiO2 films and its contribution to the reduction of hot-carrier degradation", J. Takahashi, K. Machida, N. Shimoyama, and K. Minegishi [NTT], Appl. Phys. Lett. 62 (#19) 2365 (May 1993)
5 "Enhanced Hot-Carrier Degradation Due to Water-Related components in TEOS/O3 Oxide and Water Blocking with ECR-SiO2 Film", N. Shimoyama, K. Machida, J. Takahashi, K. Murase, K. Minegishi and T. Tsuchiya [NTT], IEEE Trans El Dev ED40 1682 (1993)
6 "Improvement of Water-Related Hot-Carrier Reliability by Using ECR Plasma SiO2", K. Machida, N. Shimoyama, J. Takahashi, Y. Takashi, N. Yabumoto and E. Arai [NTT], IEEE Trans El Dev ED41 709 (1994)
7 "A Stress-Temperature Hysteresis and Gas Desorption Study of Various Oxide Films Used in Intermetal Dielectric Applications"S. Blain, L. Ouellet, Y. Tremblay [MITEL], DUMIC '95 p. 111
8 "Highly Reliable silicon Oxynitride Films formed by Biased ECR CVD for the Interlayer Dielectric of Multilevel Interconnections", H. Ishikawa and Y. Murao [NEC], VLSI Multilevel Interconnect Conference (VMIC) 1994 p. 67
9 "Moisture-Blocking Mechanism of ECR-Plasma SiO2 and High Reliable Performance of Multilevel Metallization", M. Doki, H. Watatani, S. Okuda, and Y. Furumura [Fujitsu], VMIC 1994 p. 235
10 "Moisture Trapping and Pinhole Suppression by the use of High Refractive Index PECVD SiO2 Thin Film", P. Lee, B. Pang, J.Huang, C. Ngai and D. Cheung [AMT], VMIC 94 p. 299
11 "Control of Field Threshold Voltage through Optimization of PECVD Oxide Process Conditions", J. Dun, S. Tai, D. Whiteside, D. Dang, R. Chuang, T. Tafari [Siliconix], VMIC 94 p. 320
12 "Enhanced HC Degradation Due to Hydroxyl Groups in Plasma Enhanced TEOS Oxides", T. Yamaha, Y. Inoue, t. Fujioka, O. Hanagasaki and T. Hotta [Yamaha], VMIC 95 p. 403
13 Silicon Processing for the VLSI Era, Vol. II, S. Wolf, Lattice Press 1990 [ISBM 0-961672-4-5], p. 348 ff.
14 “Water Desorption Control of Interlayer Dielectrics to Reduce MOSFET Hot Carrier Degration", K. Shimokawa, T. Usami, and M. Yoshimaru [Oki], IEICE Trans. Electronics E77C p. 473 (1994)
Selected Shorts Table of Contents