TEOS / Ozone Thermal CVD
As we discussed previously, TEOS is a relatively inexpensive, safe source for silicon dioxide. However, deposition using oxygen or inert ambients requires temperature in excess of 600 °C. To achieve lower temperature deposition, it is necessary to add a more aggressive oxidant. The use of ozone as this oxidant has been widely explored and found commercial applications.
Ozone is triatomic oxygen, O3. The molecule is metastable at room temperature, slowly degrading into molecular oxygen over a few days. The decomposition reaction, which generates monatomic oxygen intermediates, is strongly activated and takes place in milliseconds at temperatures > 200 °C. Ozone is normally generated from oxygen at the point of use, since it cannot be readily stored for long periods. Ozone in concentrations of greater than about 12-15 mole% are explosive even at room temperature, limiting most applications to maximum concentrations of less than 10 mole %. Generators use "silent discharge" (RF capacitive discharges at atmospheric pressure through a dielectric wall) cells; efficient generation requires generous cooling provisions to keep the cell temperature low, and avoid decomposition of the ozone as it is produced. Traces of nitrogen are sometimes added to help catalyze the formation of ozone and stabilize the generator output, but may generate nitric oxides and help corrode metal plumbing and contaminate wafers in process.
Ozone is highly toxic, but also has excellent warning properties -- you can smell it easily around copying machines in concentrations well below toxic levels. Toxic monitoring and handling in organic-free plumbing are essential: ozone will attack most sealing materials (o-rings) during long-term exposure. Ozone dissolves in water but does not decompose immediately, so simple water scrubbing of exhaust is not adequate for treatment; burning or catalysis are helpful.
Deposition
With the addition of a few mole% of ozone to oxygen, silicon dioxide deposition can be obtained at much lower temperatures than with oxygen alone:
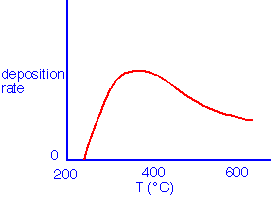
The deposition rate is generally observed to saturate as the concentration of ozone is increased, although film quality (discussed below) is often improved by using ozone concentrations well in excess of the "knee":
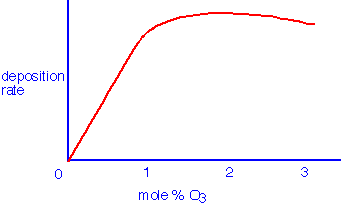
The mechanism certainly involves attack of monatomic oxygen produced in the heated gas on TEOS both at the surface and in the gas phase. Gas phase reactions have been shown to represent a significant influence on deposition in SACVD (sub-atmospheric) and APCVD processes; they are probably less important at low pressures (< 10 Torr). Typical reactions involve the attack of the alkyl group with e.g. Si-OH left behind, for example:
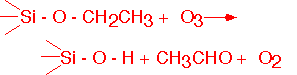
Film Quality: Moisture, Stress, and Cracking
TEOS/ozone films, like TEOS/O2 films, have excellent conformality: in some cases they even seem to display a "flow-like" or more-than-conformal behavior, filling re-entrant features that could not be filled by merely uniform deposition over the existing topography. However, this excellent behavior comes at a price.
Some of the earliest work on TEOS/ozone reactions was performed at pressures of 1-10 Torr, but it was found that film quality is much improved by deposition at higher pressures. Most commercial implementations of this process employ pressures of 200-"760" Torr. Even at higher pressures, TEOS/ozone films are subject to several problems which are rarely observed in thermal deposition from silane, or from TEOS and oxygen at high temperature. In this section we discuss the nature of some of the problems.
As we noted previously, CVD silicon dioxide is amorphous and cannot be characterized by classic crystallographic (x-ray diffraction) techniques. However, infrared spectroscopy is extremely useful: the nature and quantity of bonds in the material can be detected quickly and non-destructively. A slice of a typical IR spectrum of silicon dioxide from TEOS and ozone is shown below:
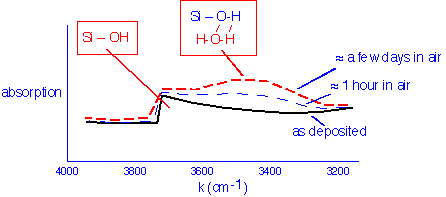
The infrared spectrum in this region is mostly sensitive to vibrations of hydrogen atoms, which due to their low mass have high characteristic frequencies. In the band shown, as-deposited films show a characteristic sawtooth absorption which is assigned to silanol (Si-OH) groups which are hydrogen-bonded to various extents to nearby silanols; the left edge of the sawtooth is near the frequency of the O-H vibration of a truly isolated silanol group, around 3750/cm. Upon exposure to air and remeasurement, we observe the growth of a more symmetrical broad absorption centered around 3300/cm. This absorption is assigned to water molecules hydrogen-bonded to the silanol groups. The extent of this symmetric absorption is essentially an indicator of the amount of water absorbed into the film from the air.
The absorption of water found in the IR spectrum is also indicated in the film stress, typically measured by the change in the curvature of the wafer without and without the film. A typical result for stress during a thermal cycle is shown schematically below:
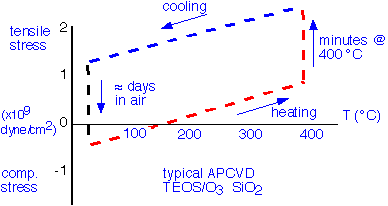
The films are under modest tensile stress as deposited, but if the measurement of stress is not performed quickly, apparent compressive stress will be measured as water is absorbed into the film over the course of a few hours. Upon heating, the water is driven off, with a consequent increase in tensile stress superimposed upon the tensile stress resulting from differential thermal expansion of the silicon wafer and silicon dioxide film. If the wafer is heated at maximum temperatures up to about 400 °C, the stress upon cooling will be more tensile than it was initially, and will slowly relax back to compressive stress over several days: this is the phenomenon of stress hysteresis.
Stress hysteresis and IR absorption both signal the presence of water molecules in the films. The water can diffuse into underlying gate oxides and degrade hot-electron reliability of transistors. The stress variations can also impact the reliability of aluminum metallization.
It seems likely (at least to me) that the underlying cause of the increased susceptibility of TEOS/ozone films to silanol incorporation is the large size of the TEOS molecule. Films deposited from TEOS must undergo significant restructuring to form dense silicon dioxide, as the "holes" left behind by the elimination of ethane or ethoxy groups are filled in. This restructuring becomes difficult if the adsorbed molecule is "under" the surface, covered by subsequent deposition. Thus, higher deposition rates (necessary to reduce cost) give poorer films. Either no bridge bonds are made, giving porous films, or the bonds are strained, and thus susceptible to later hydrolysis. Silane chemistry only requires the elimination of small hydrogen molecules (or perhaps water molecules), and doesn't lead to as much porosity; Si-H bonds incorporated into the film may also act as an "internal getter" for any residual moisture. High-temperature TEOS deposition allows bonds to stretch and readjust during deposition, and again produces a denser and purer film.
Thus in order to take advantage of TEOS/O3 step coverage without impacting transistor reliability, the TEOS/O3 film is usually encapsulated within layers of plasma-deposited oxide. Achieving uniform growth in these conditions is dependent on avoidance of another problem unique to TEOS/ozone: surface sensitivity. The deposition rate of TEOS/ozone films is strongly influenced by the nature of the substrate. Film growth is rapid and facile on bare silicon, but thermal oxide substrates produce slow-growing, porous films when deposition is performed by SACVD or APCVD at high ozone concentrations. Traces of fluorine at surfaces also have a strong deleterious effect on deposition rate; F has been intentionally introduced to improve planarization by suppressing growth over metal lines. Introduction of nitrogen on the surface by nitrogen or ammonia plasma treatments enhances growth. Growth on silicon nitride is modestly suppressed. Thus the nature of the underlayer and possible trace contamination at the surface must be considered for successful TEOS/O3 integration.
Consequences of Chemistry
Conformality and gap fill: Conformality is excellent under most conditions, and sometimes spectacular. However, the need to sandwich the TEOS/O3 film between two PECVD films can degrade the overall ability of the IMD to fill gaps. Doped films (BPSG) have poorer conformality than undoped films.
Smooth film: Very smooth surfaces are normally produced.
Silanol and stress: Films contain silanol as deposited and show stress hysteresis and moisture absorption. Higher ozone concentrations, lower deposition rates, and higher deposition temperature help.
SA/APCVD preferred: High pressures are required for usable films, presumably to increase the absolute concentration of ozone. APCVD is commonly employed; alternatively, pressures between 200 and 700 Torr in a sealed chamber may be used.
Today (2016) TEOS/ozone films have found application in coating the walls of highly-anisotropic holes drilled in silicon to provide through-silicon vias (TSV’s) for advanced packaging applications.
Some nostalgic references:
"Silicon Dioxide Deposition by Atmospheric Pressure and Low-Temperature CVD Using TEOS and Ozone" K. Fujino, Y. NIshimoto, N. Tokumasu, and K. Maeda (SPL) J Electrochem Soc 137 2883 (1990)
"Doped Silicon Oxide Deposition by Atmospheric Pressure and Low Temperature Chemical Vapor Deposition Using Tetraethoxysilane and Ozone" K. Fujino, Y. Nishimoto, N. Tokumasu and K. Maeda (SPL) J. Electrochem. Soc. 138 3019 (1991)
"Surface Modification of Base Materials for TEOS/O3 Atmospheric Pressure Chemical Vapor Deposition" K. Fujino, Y. Nishimoto, N. Tokumasu and K. Maeda (SPL) J. Electrochem. Soc. 139 1690 (1992)
"Surface Related Phenomena in Integrated PECVD/Ozone-TEOS SACVD..." K. Kwok, E. Yieh, S. Robles and B. Nguyen J. Electrochem. Soc. 141 2172 (1994)
"The Effects of Alkoxy functional Groups on Atmospheric-Pressure Chemical Vapor Deposition Using Alkoxysilane and Oxygen", K. Ikeda, S. Nakayama and M. Maeda, J. Electrochem Soc 142 1659 (1995)
"Gap Fill and Film Reflow Capability of Subatmospheric Chemical Vapor Deposited Borophosphosilicate Glass", S. Robles, K. Russell, M. Galiano, V. Siva, V. Kithcart and B. Nguyen, J. Electrochem. Soc. 143 1414 (1996)
"Tetraethylorthosilicate Vapor Treatment for Eliminating Surface Sensitivity in Tetraethylorthosilicate / O3 Atmospheric-Pressure chemical Vapor Deposition", K. Tsukamoto, D. Cheng, H. Komiyama, Y. Nishimoto, N. Takumasu and K. Maeda, Electrochemical and Solid State Letters vol 2 #1 p. 24 (1999)
Return to Tutorial Table of Contents
Book version of the CVD Tutorial